Authors: Elixirgen Scientific
Human induced pluripotent stem cells (hiPSCs) hold much promise in providing unique opportunities to advance understanding and treatment of human disease. hiPSCs can be expanded in culture indefinitely and can be differentiated into cells of all types, thereby providing a valuable source of trillions of reproducible cells for extended research. Such hiPSCs can be established from healthy or diseased individuals to study normal physiology as well as specific diseases and contribute towards drug discovery and new therapies. We have developed a rapid differentiation method, namely Quick-NeuronTM technology, resulting in the conversion of hiPSCs to highly homogenous neurons in 7 to 10 days[1] . These neurons display high levels of purity and low batch-to-batch variation and have been consistently produced from dozens of different hiPSC lines, including patient-specific lines and lines with known mutations. Our cells function comparably to their in vivo counterparts and can be used in 2D or 3D (e.g., spheroids, organoids, and organ-on-a-chip) models for the advancement of regenerative medicine or for phenotypic, and high-throughput toxicology screening of drug efficacy and toxicity.
Results
Excitatory Neurons
The Quick-NeuronTM Excitatory Neuron technology, Elixirgen Scientific’s proprietary transcription factor-based technology, allows rapid and reproducible differentiation of hiPSCs into neurons without sacrificing the purity of the cells. We have characterized our neurons using immunofluorescent staining for marker expression, RNA sequencing to look at gene expression, as well as through functional assays such as patch clamping and micro- (or multi-) electrode arrays (MEA). Our Quick-NeuronTM Excitatory Neurons exhibit typical morphology with neurite outgrowth and express a variety of neuronal markers, such as the pan-neuronal marker tubulin beta 3 class III (TUBB3) and the glutamatergic neuron marker vesicular glutamate transporter 1 (vGLUT1/SLC17A7) by day 10 of differentiation (day 7 post-thaw: Figure 1). Whole-cell patch-clamp experiments revealed spontaneous action potentials and spontaneous excitatory postsynaptic current by voltage clamp measurement at -70 mV, indicating the formation of mature synapses among neurons (Figure 2A-C). Excitatory neurons can be maintained in culture for at least 7 weeks and are suitable for a variety of applications such as neurotoxicity assays.
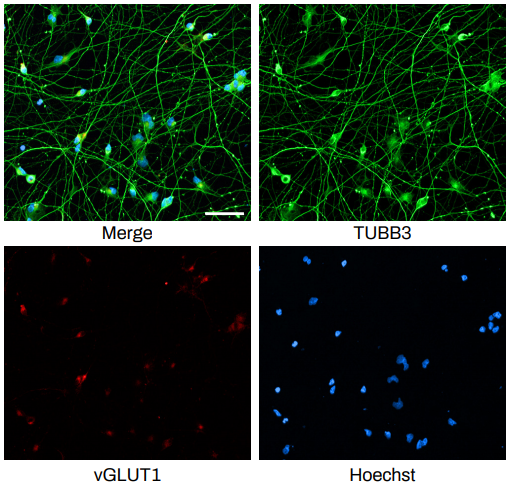
RNA-seq profiles
Human iPSCs were differentiated to excitatory neurons using our Quick-NeuronTM Excitatory Neuron technology and maintained in culture for up to 52 days. Gene expression profiling was performed at several time points by RNA-seq and similarities of gene expression profiles to human brain tissue were assessed by principal component analysis (PCA). PC1 (the x-axis) clearly separates the gene expression profile of hiPSCs from those of excitatory neurons and the human brain (Figure 2D). Progression of neural maturation is observed, such that excitatory neurons in culture for a shorter time display gene expression profiles similar to that of fetal human brain cells while cells that remain in culture for a longer period of time more closely resemble the gene expression profile of adult human brain cells. In particular, the gene expression profile of our excitatory neurons cocultured with human primary astrocytes (ThermoFisher) displayed a high similarity to that of the human brain, with markers of neurons, astrocytes, and microglia as well as markers of inhibitory neurons including GAD1/GAD67 and GAD2/GAD65 expressed (Figure 2D-E).
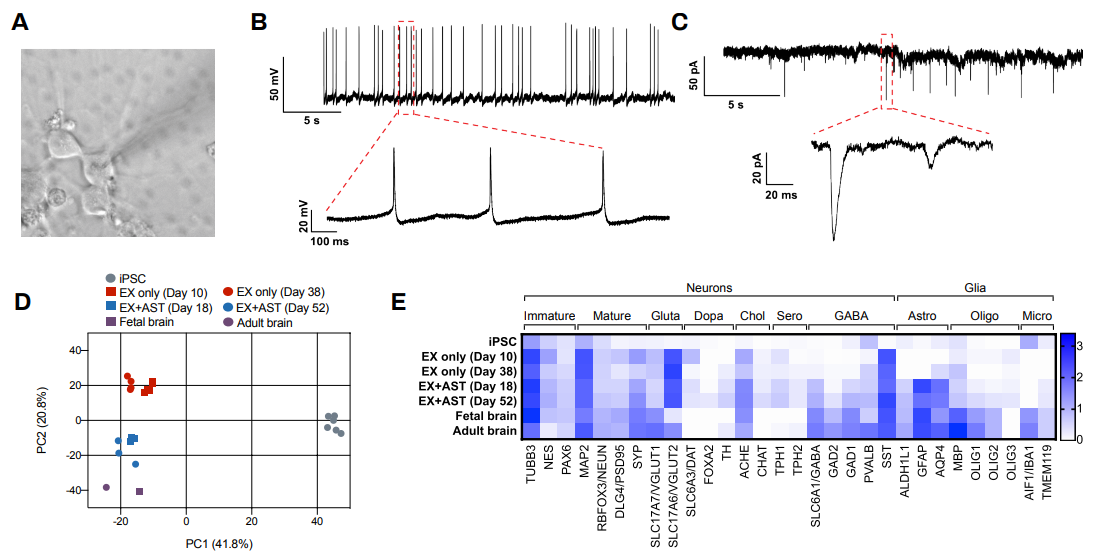
MEA-drug responses
Having established that Elixirgen Scientific’s QuickNeuronTM Excitatory Neurons display physiologically relevant markers, global gene expression, and electrophysiology, we next explored their utility in MEAs. MEAs offer a powerful, scalable, and highly sensitive platform to investigate functional networks of hiPSC-derived neurons in a high-throughput manner2 . They have been used to study neuronal function and brain disorders as well as to evaluate the toxicity of chemical compounds3-4. Elixirgen Scientific’s Quick-NeuronTM Excitatory Neurons were cocultured with primary human astrocytes on MEA plates for 51 days post-thaw, and exposed to 3 concentrations each of compounds categorized as receptor agonists, antagonists, or ion channel blockers. Quick-NeuronTM Excitatory Neurons displayed expected responses to each of the compounds (Table 1).
Acetaminophen, at concentrations of 1-100 μM, served as a negative control and resulted in no change in the spike count upon exposure. On the other hand, administration of the muscarine receptor agonist pilocarpine hydrochloride at 0.1-10 μM and the histamine receptor agonist histamine at 0.3-30 μM to the cultures each resulted in an increase in the number of spikes, indicating that excitation was induced in these neurons. In contrast, when the GABA receptor agonist GABA was applied to the cultures at 0.1-10 μM, it resulted in a decrease in the number of spikes, indicating the presence of inhibitory synapses among the network of these neurons. Interestingly, dopamine decreased the number of spikes when administered at 0.1 μM but increased it when administered at 10 μM, while glutamate had the opposite effect - causing excitement at lower dosages and suppression at a higher dosage. The AMPA/Kainate receptor antagonist CNQX suppressed neuronal activity at 0.5-50 μM, whereas GABA receptor antagonists picrotoxin and gabazine induced excitement responses in the neurons at 0.1-10 μM and 0.3-30 μM, respectively. After exposure to dopamine D2 receptor antagonist chlorpromazine and histamine receptor antagonist ketotifen, the number of spikes increased slightly at 0.1 μM but markedly decreased at 10 μM.
Similar to glutamate, the glycine antagonist strychnine increased activity at lower dosages and suppressed at the highest dosage. Antiepileptic drug carbamazepine, a sodium channel blocker, served as a suppressor of neuronal activity and, as expected, the number of spikes decreased at 10 μM. Potassium channel blocker 4-Aminopyridine (4-AP) increased the number of spikes when administered at 0.3-30 μM. Importantly, when an MEA plate seeded with Quick-NeuronTM Excitatory Neurons and primary human astrocytes and maintained for 51 days was shipped from Tokyo, Japan to Maryland, USA (a journey of 33 hours), the number of active MEA electrodes and the basal activity remained unchanged and the neurons displayed expected responses to 4-AP (Figure 3), signifying that the culture of neurons on MEA plates can be out-sourced as a service for researchers and pharmaceutical companies around the globe.
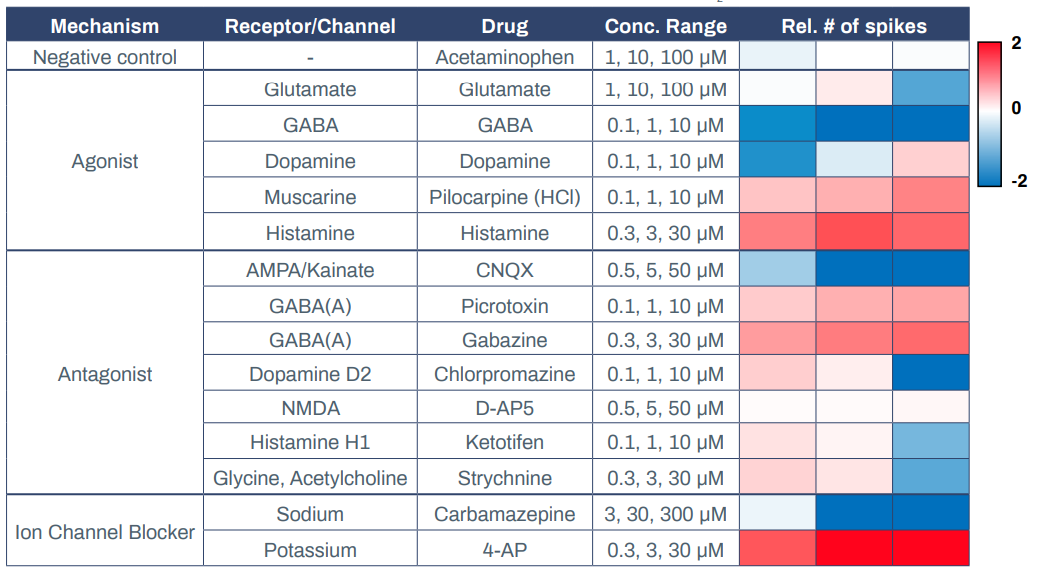
Methods
Cell Culture
Elixirgen Scientific’s Quick-NeuronTM Excitatory Neurons (Catalog No. EX-SeV-CW50065) were thawed and maintained following the instructions laid out in our user guide.
Patch Clamp
Quick-NeuronTM Excitatory Neurons were thawed and maintained long-term as instructed by our user guide for 5-6 weeks prior to patch clamp. Spontaneous synaptic activity and action potentials were measured by E-PHY SCIENCE SAS.
RNA Sequencing
Quick-NeuronTM Excitatory Neurons were lysed for total RNA extraction on days 7 and 35 after recovery from cryopreservation (i.e., days 10 and 38 after differentiation induction). Quick-NeuronTM Excitatory Neurons were also co-cultured with primary human astrocytes (ThermoFisher, Catalog No. N7805200) on an MEA plate and lysed for total RNA extraction on days 15 and 49 after plating (i.e., days 18 and 52 after differentiation induction).
Total RNA was processed using the SMART-seq2, followed by the library preparation using the Nextera XT DNA library preparation kit. Paired end sequences of 75 bp were obtained using NextSeq 550 (Illumina) up to 20 million reads. Fastq file was used to map sequences on the human reference genome assembly 38 after removing low quality reads. The levels of gene expression were expressed as the number of transcripts per million transcripts (TPM).
Microelectrode array
Quick-NeuronTM Excitatory Neurons were plated at 0.8 x 10⁵ cells/well of a 48-well MEA plate with 16 electrodes per recording area (1.21 mm2; Axion Biosystems), after mixing with primary human astrocytes (ThermoFisher, Catalog No. N7805200) at 0.2 x 10⁵ cells/well and 0.2 μg/ well laminin according to our application protocol. Cultures were maintained for 49 days with medium changes twice a week. Plates were incubated at 37°C, 5% CO2 . Cells in each well were exposed to a diluent of drugs (i.e., 0.1 % DMSO) first and then drugs in 3 increasing doses. Data were obtained immediately after administration of drugs using either Maestro Pro or Maestro 1 (Axion Biosystems) and collected with a sampling rate of 12.5 kHz at each electrode. After spike firing was recorded, treated cells were then washed with medium and incubated overnight. Finally, spontaneous spike firing activity was measured again to confirm that cells were not damaged by the treatment. Drug response was evaluated by measuring spike counts. Data were extracted by AxIS Navigator 2.0.4 and analysis was performed using Neural Metric Tool.
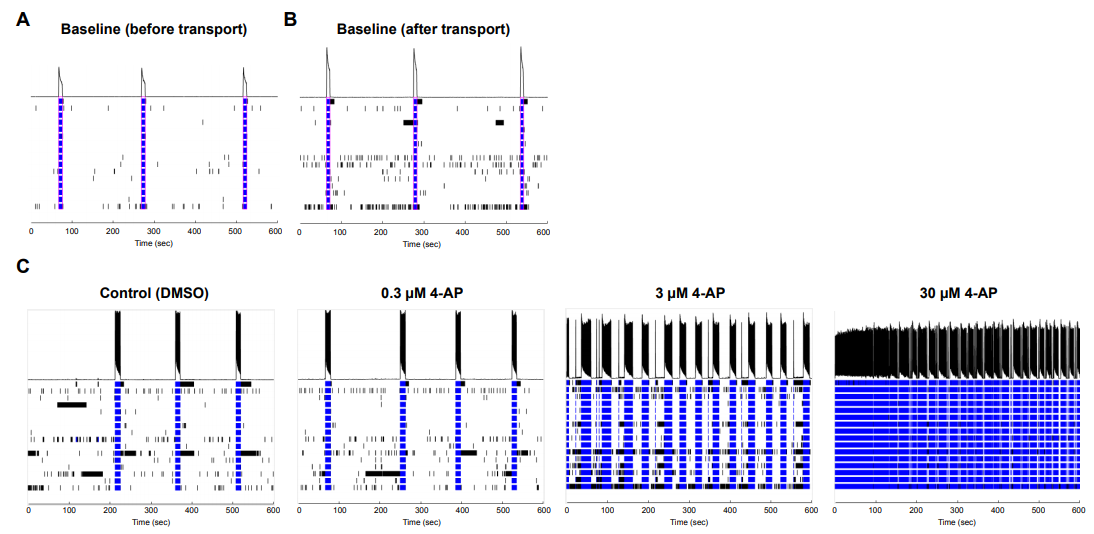
Discussion
Animal models have not fully been able to predict human drug response. Attrition of drugs due to neurotoxicity and central nervous system problems is a serious and expensive problem for pharmaceutical companies. hiPSC-derived neurons possess great potential to establish human cell-based in vitro platforms to screen out toxic small compounds, and may serve as better alternatives for the study of drug efficacy and toxicity[4-7]. Furthermore, in vitro models developed using patient-derived hiPSCs with known mutations associated with specific diseases may allow for prediction of patients’ response to treatments and therapies. The results presented here demonstrate that Elixirgen Scientific’s hiPSC-derived Quick-NeuronTM Excitatory Neurons are physiologically relevant, have gene expression profiles similar to their in vivo counterparts, and may be used for toxicity screening, pharmacogenomics, drug discovery, disease modeling, and regenerative medicine.
References
- Goparaju, S. K. et al. Rapid differentiation of human pluripotent stem cells into functional neurons by mRNAs encoding transcription factors. Sci. Rep. 7, 1–12 (2017).
- Yuan, X. et al. Versatile live-cell activity analysis platform for characterization of neuronal dynamics at single-cell and network level. Nat. Commun. 11, (2020).
- Kim, B. W. et al. Human Motor Neurons With SOD1-G93A Mutation Generated From CRISPR/Cas9 Gene-Edited iPSCs Develop Pathological Features of Amyotrophic Lateral Sclerosis. Front. Cell. Neurosci. 14, 1–16 (2020).
- Odawara, A., Matsuda, N., Ishibashi, Y., Yokoi, R. & Suzuki, I. Toxicological evaluation of convulsant and anticonvulsant drugs in human induced pluripotent stem cell-derived cortical neuronal networks using an MEA system. Sci. Rep. 8, 1–11 (2018).
- Tukker, A. M., van Kleef, R. G. D. M., Wijnolts, F. M. J., de Groot, A. & Westerink, R. H. S. Towards animal-free neuro- toxicity screening: Applicability of hiPSC-derived neuronal models for in vitro seizure liability assessment. ALTEX 37, 121–135 (2020).
- Tukker, A. M., Wijnolts, F. M. J., de Groot, A. & Westerink, R. H. S. Human iPSC-derived neuronal models for in vitro neurotoxicity assessment. Neurotoxicology 67, 215–225 (2018).
- Grainger, A. I. et al. In vitro models for seizure-liability testing using induced pluripotent stem cells. Front. Neurosci. 12, 1–14 (2018).