By Jim Ross, PhD
European Biopharmaceutical Review, 2022.
Alongside the herculean efforts the pharmaceutical industry has undertaken to get vaccines rolled out across the globe, identifying safe and effective drugs to treat COVID-19 will be crucial in the struggle to get to grips with the virus
COVID-19 is continuing to spread around the world, with roughly 300 million confirmed cases and more than five million deaths (1). Health officials cite numerous causes for this ongoing threat, including wavering vaccine supply and demand, the emergence of new variants, and a lack of effective therapeutics for the disease. Although mitigation efforts are ongoing, epidemiologists predict the likeliest long-term outcome is that the SARS-CoV-2 virus will become endemic in large regions around the world, constantly circulating among the human population (2). To help reduce the impact of COVID-19 on global health, any long-term management strategy must include the creation of safe and effective antiviral drugs.
Identifying a COVID-19 Treatment Strategy
Historically, scientists have found it difficult to develop antiviral drugs for several reasons. Firstly, viruses only contain the genetic material and proteins they need to travel through cell membranes and hijack a cell’s replication machinery. As a result, they do not possess many ideal drug targets. Also, because viruses rely so much on cells to operate, it is challenging to develop a drug that neutralises the virus without interfering with normal physiological processes. Furthermore, viruses mutate frequently, making it hard to identify a stable target that will be useful over a long period of time. In fact, although SARS-CoV-2 was only discovered two years ago, scientists have already identified highly contagious variants that may result in more severe and/or transmissible disease and threaten the efficacy of today’s vaccines. Consequently, ideal antiviral drugs must target a portion of the virus or a related process that does not change. Most antiviral drugs used today either prevent viruses from entering cells or block their replication. Chris Basler, PhD, of the Icahn School of Medicine at Mount Sinai, found that one promising method of stopping SARS-CoV-2 might involve the latter approach. Fortunately, coronaviruses exhibit a unique, stable, and critical behavior during replication, which antiviral developers can exploit to treat the infection. To replicate in a host cell, coronaviruses reorganise and recruit host cell membranes to form replication organelles. Researchers have found that a drug targeting these organelles may be able to inhibit coronavirus replication and reduce the severity of an infection (3). Dr Basler and his team, while at Georgia State University, assessed the effect of various therapeutics on the cell-killing (cytopathic) behaviour of SARS-CoV-2 using a bioelectronic assay (4).
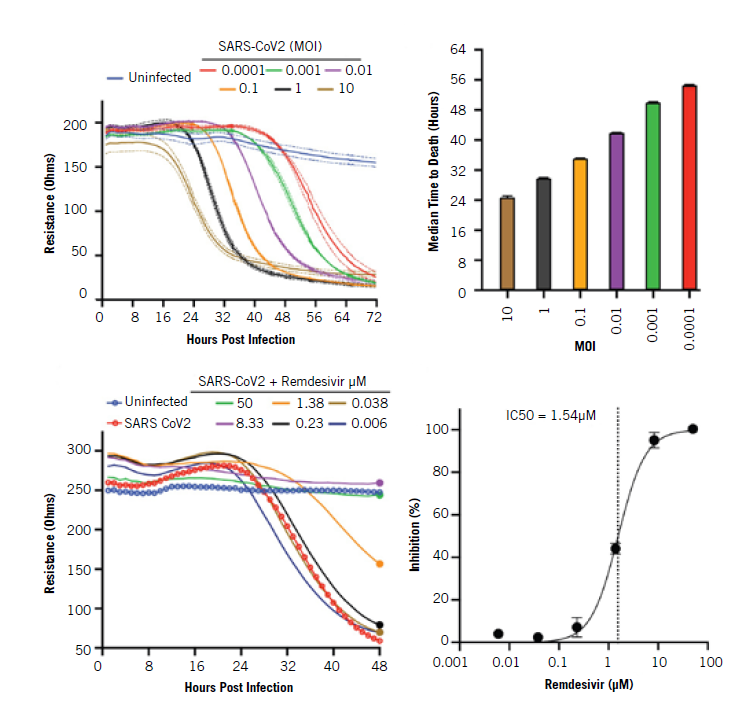
Using an Impedance-Based Bioelectronic Assay to Measure Viral Activity
One way to measure the virulence of a virus is to measure cell death upon infection. Bioelectronic assays, which use multiwell plates with multielectrode arrays (MEAs) embedded in the bottom of each well, measure cell death noninvasively, and in real time, using electrical impedance (5). As each well sends a small electrical signal across the bottom of a well, the assay measures the extent to which the contents of the well impede, or obstruct, the signal. The health and integrity of the cells are indicated by the level of impedance, which rises as cells attach and grow, and falls as the cell membrane destabilises and the cells die and detach.
Bioelectronic assays can monitor small cellular changes in real time across minutes or days. This continuous monitoring is useful for tracking a virus’ cytopathic effects when its kinetics vary or is unknown. Furthermore, unlike many cell-based assays that require the use of dyes or labels, which can impair cellular health or create background noise that reduces the sensitivity of the assay, bioelectronic assays require no additional reagents and can reveal variations below the detection limit of other techniques.
Since they require no additional reagents, bioelectronic assays are also simple to run and amenable to higher throughput testing. Easily quantifiable electrical signals provide a clear measure of cell death, making analysis straightforward. Because of these advantages, Dr Basler used a bioelectronic assay to assess the magnitude and kinetics of SARS-CoV-2-induced cell death in response to various therapeutic candidates.
Bioelectronic Assay Validation
Dr Basler’s team used the bioelectronic assay at multiple steps in the process, beginning with an examination of the impact of SARS-CoV-2 on healthy cells. They first plated Vero E6 cells on an MEA plate and allowed them to attach in each well. Then, they incubated the plates under physiological conditions and added the virus at various concentrations. Multiplicities of infection (MOI), the ratio of virus particles to cells, varied from 0.0001 to 10, and they measured how each culture responded every minute over the course of 72 hours.
At the highest MOIs of 10 and 1, the virus led to a decrease in impedance within a day, while at the lowest concentration, cells began to die after only two days. All MOIs led to maximal cell death between 32 and 72 hours.
The researchers also tested whether the assay could detect any treatment effect from remdesivir, a drug that inhibits SARs-CoV-2 infection. They calculated percent inhibition and found that the drug reduced the impact of SARS-CoV-2 on the cells at the same rate that it did in previous studies using plaque assays. These results demonstrated that the assay was effective at measuring both the dynamic impact of SARS-CoV-2 on cells as well as the inhibitory effect of therapeutics. Using the validated assay, Dr Basler’s team then tested the ability of novel drug candidates to reduce the impact of SARS-CoV-2 on healthy cells in vitro.
The Methodology Behind Testing Novel Compounds Against SARS-CoV-2
As described previously, SARS-CoV-2 recruits host cell membranes to form replication organelles. Knowing this, Dr Basler’s team assessed compounds that target processes involved in fatty acid metabolism and cell membrane biology. They first examined inhibitors of VPS34, a class III phosphoinositol-3 kinase that scientists have found to contribute to the replication of other viruses such as tombusvirus and hepatitis C.
They applied two VPS34 inhibitors, VPS34-IN1 and PIK-III, to the wells of their plate at ten different concentrations and measured impedance every minute for 72 hours.
The assay enabled the researchers to observe that the lowest concentrations inhibited cell death in a dosedependent manner and that the two highest doses were toxic.
Using the same assay, the researchers found that the fatty acid metabolism inhibitors orlistat and triacsin C also prevent virus-induced cell death. The team did not see the same toxic effects at higher concentrations that were observed with the VPS34 inhibitors. Together, these results indicated new target mechanisms for therapeutic development against SARS-CoV-2 virus.
Bioelectronic Assay Use Cases and Benefits
Leveraging bioelectronic assays to explore potential new antiviral targets for SARS-CoV-2 merely scratches the surface of this technology’s potential (5). As explored above, bioelectronic assays can track cell proliferation, morphology, and viability label-free and in real time. They are ideal for immunooncology, cytotoxicity, cell migration, cell proliferation, and GPCR assays. Additionally, bioelectronic assays are suitable for virology applications, such as measuring viral titres, screening the fitness of viral variants, and detecting the presence and effectiveness of neutralising antibodies.
Bioelectronic assays enhance our ability to quickly study interactions between cells, viruses, and therapeutics in real time over days, in one plate, without interference by labels or tags. The user-friendly, high-throughput, in vitro system allows the researcher full environmental control. Requiring only basic cell culture techniques, the continuous recording capabilities of the assay enable scientists to capture complex culture dynamics in a single plate in short- or long-term experiments.
These assays open the door to more nuanced antiviral screening. Measuring in real time not only allows scientists to discover ideal doses of potentially effective antiviral treatments, but also enables the identification of toxic or ineffective treatments early in the development process before animal testing begins.
Looking ahead, Dr Basler and his team are optimistic that the new discoveries made possible by this technology will lead to more effective therapies for challenging viruses such as SARS-CoV-2 and make an impact on our future approach to tackling life-threatening diseases.
References
- Visit: covid19.who.int
- Visit: www.nationalgeographic.com/science/article/ covid-19-will-likely-be-with-us-forever-heres-how-welllive- with-it
- EJ Sneijder et al, A unifying structural and functional model of the coronavirus replication organelle: Tracking down RNA synthesis, PLOS Biology: pp1-2, 2020
- CG Williams et al, Inhibitors of VPS34 and fatty-acid metabolism suppress SARS-CoV-2 replication, Cell Reports: pp2, 2020
- Visit: www.axionbiosystems.com/resources/news/ bioelectric-assay-identifies-potential-covid-19- therapeutic-targets
Jim Ross, PhD, is the CTO and co-founder of Axion BioSystems as well as the co-founder and scientific advisor of BioCircuit. Dr Ross has over twenty years’ experience in bioelectronic technology development, devoting much of his efforts to commercialising cost-effective cellular interfaces and softwarereconfigurable analysis tools.
Dr Ross received his undergraduate degree in electrical engineering from Louisiana State University, US, in 2000 and his PhD in Neuroengineering from the Georgia Institute of Technology, US, in 2008.
Dr Chris Basler receives compensation as a paid consultant for Axion BioSystems.